This study proposes a novel system to monitor the pH characteristics and electrical conductivity of 3-Hydroxy-3-methylhexanoic acid and 3-mercapto-3-methyl-1-hexanol, which are causative agents of underarm odor, to estimate underarm odor. A multimodal sensor, which can measure electrical conductivity, temperature, and pH, and an AgCl external electrode were adopted in the system to measure the pH of the agents on the skin. Experiments were conducted to measure the accuracy of the electrical conductivity and the pH for 3-hydroxy-3-methylhexanoic acid and 3-mercapto-3-methyl-1- hexanol to verify the applicability of the proposed system. The results showed that the proposed system may be able to accurately measure the pH and electrical conductivity of the causative agents.
Underarm odor results from a combination of odorous substances that are produced by common bacteria, such as colynebacteria and staphylococci, which are located in the apocrine sweat glands, and other indigenous bacteria [1, 2]. Although underarm odor is dominant worldwide, East Asians such as Japanese, Chinese, and Korean with underarm odor constitute a minority of approximately 10% [3]. Strong body odor is thus generally considered to be a disease in Japan. In recent years, the fat intake of the Japanese population has increased with the introduction of Western food, which will encourage the development of apocrine sweat glands and an increase in the number of Japanese patients with underarm odor.
There is no diagnostic guideline for underarm odor in Western countries because underarm odor is not considered to be a disease but a physiological phenomenon. However, there are several approaches for the diagnosis of underarm odor in Japan: (1) confirmation of apocrine glands by incision, (2) presence of wetness of earwax, (3) family history of odor odors, and (4) judgment by sense of smell of specialist [4]. Underarm odor is considered to be asymptomatic because human smell adapts to a constant odor. That is, a human becomes insensitive to his underarm odor. Therefore, a system that can easily detect underarm odor will be useful for self-diagnosis.
Some researchers have proposed a monitoring system for body odors [5-11]. However, to the best of the authors’ knowledge, there are no reports on the diagnosis of underarm odor by utilizing these sensors. 3-Hydroxy-3-methylhexanoic acid and 3-mercapto- 3-methyl-1-hexanol are known as causative agents of underarm odor [12, 13]. A detection device for underarm odor will be able to measure these characteristics accurately.
To this end, this study proposes a novel monitoring system for pH characteristics and electrical conductivity (EC) of 3-Hydroxy-3-methylhexanoic acid and 3-mercapto-3-methyl-1-hexanol for simple estimation of underarm odor.
Futagawa et al. proposed a multi-modal sensor chip that is integrated with an EC sensor using a Pt electrode, a temperature sensor using a p-n junction diode, and a pH sensor using an ion-sensitive FET, utilizing Si LSI technology, as shown in Figure 1 [14, 15]. This sensor chip is small, with a size of 5 mm × 5 mm, and undergoes electric insulating treatment to facilitate the simultaneous measurement of electrical impedance, pH, and temperature of soil. Figure 2 shows the measuring circuit of the EC. The EC σ is measured from the voltage and current that flows between the two electrode terminals shown in Figure 1 and is calculated using the following equation [16]:
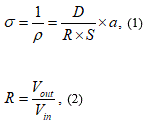
Figure 1. Overview of multi-modal sensor chip.
Figure 2. Equivalent circuit for proposed EC measurement system.
where R is the resistance of the EC inter-electrode, S is the area of the electrode, D is the inter-electrode distance, and a is a correction factor for facing electrodes and electrodes placed side by side. The values of S, a are set as 3.2 mm2 (= 4 mm × 0.8 mm), respectively, as following the previous study [16]. An AC voltage of 10 kHz is adopted as the voltage source for the measuring circuit so as to ignore the parasitic impedance between the Pt electrode and fluid interface associated with the electric double layer.
The pH scale defines the acidity or basicity of a solution based on the concentration of hydrogen ions. In a previous study, a source follower circuit using an ion-sensitive field-effect transistor (ISFET) [17] was adopted as the pH measuring sensor chip. This was because a source follower circuit has a constant gain characteristic and a superior output buffer. The pH is measured based on the change in the electrical potential of the sensitive membrane of the ISFET, corresponding to the gate voltage of the FET. The electrical potential of the solution is used for this, according to the following, Nernst equation [18]

where, VG is the electrical potential of the sensitive membrane of the ISFET, and Vre f is the electrical potential of the solution, which is given by the reference electrode, respectively. The relationship between the output voltage of the pH sensor Vout and the pH of the solution is thus given as follows:

where, α = Vout=VG is the constant gain of the source follower circuit. Generally, the reference voltage is applied to the glass reference electrode to measure the pH of the solution [19]. However, it would be difficult to measure the pH of sweat on human skin using the glass reference electrode because it needs to be immersed in the target solution. Therefore, the authors used a leakless AgCl reference electrode (LF-1, eDAQ) instead of the glass reference electrode. The AgCl reference electrode was used because there is little danger of the electrode breaking during measurement, unlike the glass reference electrode, i.e., the measuring circuit will be safe to use. Furthermore, it is assumed that the Cl contained in the sweat will prolong the life of the AgCl reference electrode. Figure 3 shows the proposed pH measurement system using the ISFET and the AgCl reference electrode.
Figure 3. Equivalent circuit for proposed pH measurement system.
The EC and the pH measurement accuracy for 3-hydroxy-3-methylhexanoic acid and 3-mercapto-3- methyl-1-hexanol (henceforth referred to as material A and B, respectively) were examined in the experiment to verify the utility of the proposed system. The odor concentration thresholds for materials A and B at which a human feels uncomfortable are reported to be 1 ng and 10 ng, respectively [13].
In the first experiment, the EC characteristics of the solutions were measured at different dilution rates using the proposed system. A commercial EC sensor (LAQUA ES-71, Horiba) was used for verification. In the second experiment, the pH characteristics of the solutions were measured at different concentrations using the proposed system, and a commercial pH sensor (LAQUA twin-pH-11B, HORIBA) was used for verification. In the preliminary experiment, the sensitivity of the pH sensor was corrected for four standard pH solutions, with pH = 4.01, 6.86, 9.18, and 10.01 in the preliminary experiment.
The second experiment involved measuring the pH values when materials A and B were applied to commercial chicken meat, which served as a phantom model of human skin. Materials A and B were applied to a portion measuring 2 cm × 2 cm × 2 cm to verify the usefulness of the proposed system. This is shown in Figure 4. The surface conditions on the phantom along the red broken line were set as follows: (i) a drop of aqueous solution included in the materials, (ii) a surface that was wetted well with the aqueous solutions, and (iii) a dried surface with the aqueous solutions. In these experiments, we investigated the relationship between each pattern and the pH characteristics by evaluating the difference in output voltage of the proposed system, because the output voltage corresponds to the pH value. The voltage applied to the reference electrode ranged from -0.5 V to 0.5 V, and the source voltage was set at 3.4 V. The output voltage V out was stored on a computer via an A/D converter, at a sampling frequency of 1 Hz.
Figure 4. Experimental setup for measuring pH of odorous material on phantom model of skin
Figure 5 shows the measured results of the EC characteristics for materials A and B using the proposed sensor. The same EC values for materials A and B were obtained using the commercial EC sensor. The results indicated that the EC of material A differed by one order of magnitude from that of material B. The EC values of both material A and material B did not change with an increase in dilution rate within the range of 10−10 – 10−5. These results indicate that the EC values may not be useful to distinguish underarm odor, because the dilution rates of materials A and B for the odor concentration thresholds are 10−10 wt%.
Figure 5. EC characteristics of (a) material A, and (b) material B
Figures 6 and 7 show the pH characteristics of materials A and B measured with the proposed system. The results show that the pH of material A changed with the concentration of its aqueous solution, whereas that of material B was constant and unrelated to the concentration. Both materials A and B exhibited acidity for all dilution rates. The pH values of materials A and B corresponding to the odor threshold were 5.0 and 4.9, respectively. Therefore, a human may perceive an odor when the pH value of a solution containing odorous materials is 5 or lower. Figure 7 shows the output voltages Vout measured for patterns (i)–(iii), as described in section. The results indicated that the gradient of (i) was different from those of (ii) and (iii). This is because the reference electrode voltage may not be applied to the solution. It is therefore concluded that it will be difficult to monitor the odorous material if sufficient material is applied to the sensor, and that the odorous material can be monitored irrespective of its drying condition.
Figure 6. pH characteristics of materials A and B
Figure 7. Comparison of output voltages Vout for each phantom
To facilitate the easy estimation of underarm odor, this study proposed a novel system to monitor the EC and pH characteristics of materials that cause underarm odor. The results indicated that the proposed system can accurately measure these characteristics of odor causing materials. In the future, it is necessary to further improve the sensitivity of this sensor, so that small amounts of odorous materials can be detected.
- Taylor D, Daulby A, Grimshaw S, James G, Mercer J, et al. (2003) Characterization of the microflora of the human axilla. Int J Cosmet Sci 25: 137-145. [Crossref]
- James AG, Hyliands D, Johnston H (2004) Generation of volatile fatty acids by axillary bacteria. International Journal of Cosmetic Science 26: 149-156.
- Martin A, Saathoff M, Kuhn F, Max H, Terstegen L, et al. (2010) A functional ABCC11 allele is essential in the biochemical formation of human axillary odor. J Invest Dermatol 130: 529-540. [Crossref]
- Japan Society of Plastic and Reconstructive Surgery, Japan Society for Surgical Wound Care, and Japan Society of Cranio-Maxillo-Facial Surgery (2015). Clinical Guidelines of Plastic Surgery. 7. KANEHARA, Tokyo.
- Persaud K, Dodd G (1982) Aanalysis of discrimination mechanisms of the mammalian olfactory system using a model nose. Nature 299: 352-355.
- Ikegami A, Arita H, Iwanaga S, Kaneyasu M (1983) Thick film sensors and their integration. Proceedings: 4th European Hybrid Microelectronics Conference Pg: 211.
- Ballantine DS, Rose SL, Grate JW, Wohltjen H (1986) Correlation of surface acoustic wave device coating responses with solubility properties and chemical structure using pattern recognition. Analytical Chemistry 58: 3058-3066.
- Stetter JR, Jurs PC, Rose SL (1986) Detection of hazardous gases and vapors: Pattern recognition analysis of data from an electrochemical sensor array. Analytical Chemistry 58: 860-866.
- Carey WP, Beebe KR, Kowalski BR, Illman DL, Hirschfeld T, et al. (1986) Selection of adsorbates for chemical sensor arrays by pattern recognition. Analytical Chemistry 58: 149-153.
- Branca A, Simonian P, Ferrante M, Novas E, Negri RM, et al. (2003). Electronic nose based discrimination of a perfumery compound in a fragrance. Sensors and Actuators B: Chemical 92: 222–227.
- Ehret B, Safenreiter K, Lorenz F, Biermann J (2011) A new feature extraction method for odour classification. Sensors and Actuators B: Chemical 158: 75-88.
- Hasegawa Y, Yabuki M, Matsukane M (2004) Identification of new odoriferous compounds in human axillary sweat. Chem Biodivers 1: 2042-2050. [Crossref]
- Natsch A, Derrer S, Flachsmann F, Schmid J (2006) A broad diversity of volatile carboxylic acids, released by a bacterialaminoacylase from axilla secretions, as candidate molecules for the determination of human-body odor type. Chem Biodivers 3: 1-20.
- Futagawa M, Iwasaki T, Murata H, Ishida M, Sawada K, et al. (2012) A miniature integrated multimodal sensor for measuring pH, EC and temperature for precision agriculture. Sensors (Basel) 12: 8338-8354. [Crossref]
- Futagawa M, Uemura K, Nomiyama K, Hirano H, Watanabe M, et al. (2018) Study of a semiconductor type ph sensor to measure low water content soils. IEEJ Transactions on Sensors and Micromachines 138: 1-9
- Futagawa M, Iwasaki T, Noda T, Takao H, Ishida M, et al. (2009) Miniaturization of electrical conductivity sensors for a multimodal smart microchip. Japanese Journal of Applied Physics 48: 04C184–1–4.
- Bergveld P (1970) Development of an ion-sensitive solid-state device for neurophysiological measure133 ments. IEEE Trans. Biomed. Eng BME 17: 70-71.
- Levine S, Smith AL (1971) Theory of the differential capacity of the oxide/aqueous electrolyte interface. Discussions of the Faraday Society 52: 290-301.
- Golan R, Gavrieli I, Lazar B, Ganor J (2014) The determination of ph in hypersaline lakes with a conventional combination glass electrode. Limnology Oceanography: Methods 12: 810–815.